Car batteries are the unsung heroes of the modern automobile. They deliver the critical power needed to start your engine, keep your electronics running, and in the case of electric vehicles, propel your ride. But have you ever wondered what’s inside this seemingly mundane box that powers your car? Let’s break down what car batteries are made of, from their chemistry to the materials that make them tick.
The Core Components of a Car Battery
While there are different types of car batteries, most fall into two categories: lead-acid batteries (used in traditional cars) and lithium-ion batteries (common in electric vehicles, or EVs). Here’s a closer look at their internal structure and materials:
1. Lead-Acid Batteries
The classic lead-acid battery is the backbone of internal combustion engine vehicles. Despite being over 150 years old, this technology remains dominant due to its reliability and affordability.
Key Materials:
- Lead Plates: Lead is the star of the show, used for the battery’s positive and negative plates. Lead plates are crucial because they participate in chemical reactions that generate electricity.
- Lead Dioxide (PbO2): This material is coated on the positive plates. During discharge, it reacts with sulfuric acid to produce electricity.
- Sulfuric Acid: The electrolyte in lead-acid batteries, sulfuric acid enables the flow of ions between the plates, allowing the battery to store and release energy.
- Plastic Casing: The outer shell of a lead-acid battery is typically made of polypropylene, a durable plastic that protects the internal components from physical damage and leakage.
- Separator Materials: Thin insulating layers between the positive and negative plates prevent short circuits while allowing ions to flow.
2. Lithium-Ion Batteries
For electric vehicles and hybrids, lithium-ion (Li-ion) batteries are the go-to choice. They’re lighter, pack more energy, and last longer than lead-acid batteries, making them ideal for modern automotive needs.
Key Materials:
- Lithium: The lightest metal and a key player in the battery’s energy density. Lithium ions move between the anode and cathode during charging and discharging.
- Cathode Materials: Often made from lithium compounds like lithium cobalt oxide (LiCoO2), lithium iron phosphate (LiFePO4), or nickel manganese cobalt (NMC). These materials determine the battery’s capacity and voltage.
- Graphite (Anode): The anode is typically made from graphite, which provides a stable host structure for lithium ions during charging.
- Electrolyte: A liquid or gel-like substance that allows lithium ions to flow between the cathode and anode. It’s often made from lithium salts dissolved in an organic solvent.
- Separator: A thin polymer membrane prevents direct contact between the cathode and anode, avoiding short circuits while permitting ion flow.
- Casing: Made of metal or durable plastic, it houses the battery and protects it from external damage.
Additional Materials and Innovations
Modern battery designs often include enhancements to improve performance, durability, and safety:
- Nickel and Cobalt: Common in lithium-ion batteries, these materials boost energy density and longevity but are costly and often scrutinized for environmental impact.
- Silicon Anodes: A cutting-edge development in lithium-ion batteries, silicon anodes can store more lithium ions than traditional graphite, increasing energy capacity.
- Thermal Management Systems: EV batteries often feature cooling mechanisms to prevent overheating and extend lifespan.
Environmental and Ethical Considerations
As essential as car batteries are, their production and disposal raise important environmental and ethical questions.
- Mining of Raw Materials:
- Materials like lithium, cobalt, and nickel are extracted through mining processes that can disrupt ecosystems and exploit labor in developing regions.
- Recycling:
- Lead-acid batteries are one of the most recycled products globally, with over 99% of components being reusable.
- Lithium-ion battery recycling is still developing but holds promise for recovering valuable materials and reducing waste.
- Sustainability:
- Manufacturers are exploring alternatives like solid-state batteries and other chemistries that use less cobalt or none at all to address supply chain and environmental concerns.
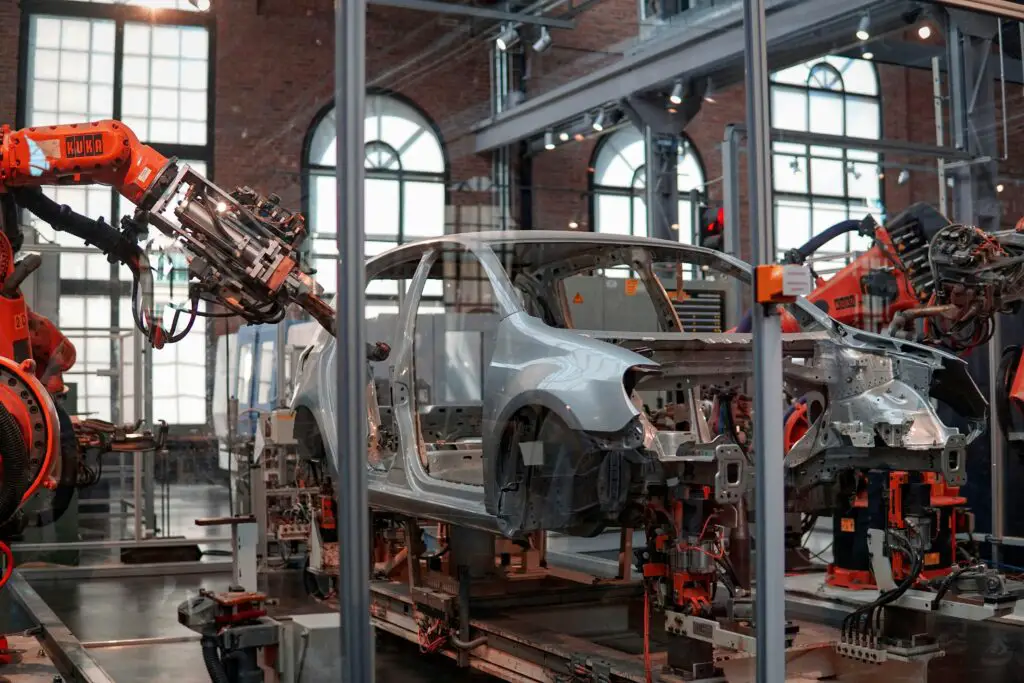
Why the Materials Matter
The materials in car batteries directly influence their performance, cost, lifespan, and environmental footprint. For instance:
- Lead-acid batteries are durable and inexpensive but heavy and less energy-dense.
- Lithium-ion batteries are lightweight and efficient but come with a higher price tag and ethical concerns over raw material sourcing.
Understanding these differences helps consumers make informed decisions about the type of vehicle or battery technology that suits their needs and values.
The Future of Car Batteries
The industry is constantly innovating to create batteries that are lighter, more powerful, and environmentally friendly. Technologies like solid-state batteries and alternatives to lithium-ion chemistries (such as sodium-ion batteries) are already on the horizon.
These advancements promise to reshape how we power our vehicles, making them more sustainable and efficient while addressing the challenges posed by traditional materials.
Final Thoughts
Car batteries might seem like simple boxes of energy, but their internal makeup is a fascinating interplay of chemistry, engineering, and materials science. Whether it’s the tried-and-true lead-acid battery under the hood of a gas-powered car or the cutting-edge lithium-ion battery driving the future of EVs, understanding what’s inside these powerhouses gives us a better appreciation for their role in modern transportation.
The next time you turn your key or push a button to start your car, remember the complex and carefully designed materials that make it all possible.
Discover more from Chikwem
Subscribe to get the latest posts sent to your email.